The start of lactation is associated with a sudden increase in calcium demand from approximately 30 g per day in late gestation to in excess of 50 g per day in some cows (DeGaris and Lean, 2008). This increase in demand can exceed the capacity of rapid-response calcium pools and calcium homeostasis mechanisms may fail, resulting in hypocalcaemia. Postpartum hypocalcaemia is one of the most well-known conditions of dairy cattle, with clinical hypocalcaemia having been described for hundreds of years (McGuffey, 2017); however, it is now recognised that subclinical hypocalcaemia is the more common form, affecting up to 60% of postpartum dairy cows (Reinhardt et al, 2011; Rodríguez et al, 2017; Venjakob et al, 2017; Neves et al, 2018).
Hypocalcaemia most commonly presents in the first three days after calving and, although any cow may be affected, high producing dairy cows in their third lactation or greater and Channel Island breeds are at higher risk. Metabolic alkalosis and hypomagnesaemia can also predispose cows to hypocalcaemia because of the negative impact these conditions have on parathyroid hormone (PTH) action (Goff, 2008). This article focusses on post-calving hypocalcaemia, but hypocalcaemia is not always associated with calving. In particular, hypocalcaemia is a common comorbidity in diseases where endotoxaemia is a feature, for example coliform mastitis (Hisaeda et al, 2020); therefore, it is worthwhile including calcium administration in treatment protocols for these conditions.
Calcium metabolism
Calcium is an important mineral involved in the structure and healthy functioning of many bodily materials and processes (Figure 1); blood calcium is tightly regulated and maintained at a consistent concentration of 1.5‒2.0 mmol/L (Goff, 2008). The parathyroid gland is fundamental to calcium metabolism, with bone, the kidneys and the gastrointestinal tract being primary target organs responsible for maintaining calcium homeostasis.
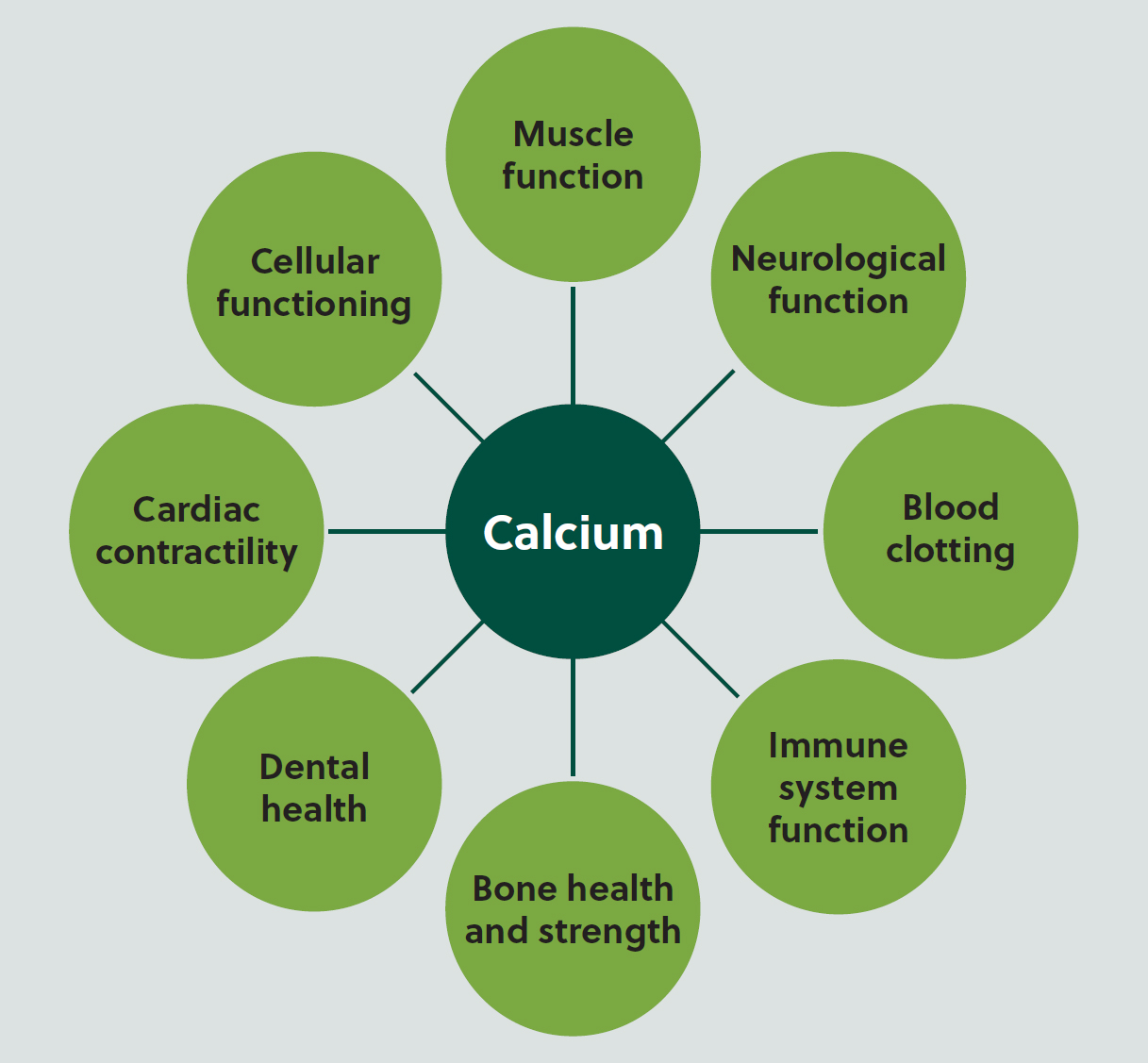
Parathyroid hormone, calcitonin and vitamin D are the principal hormones that regulate calcium concentration: PTH responds to decreases in calcium and calcitonin responds to increases in calcium in a negative feedback loop that ensures calcium concentration remains stable (Figure 2) (Petroff and Greco, 2021).
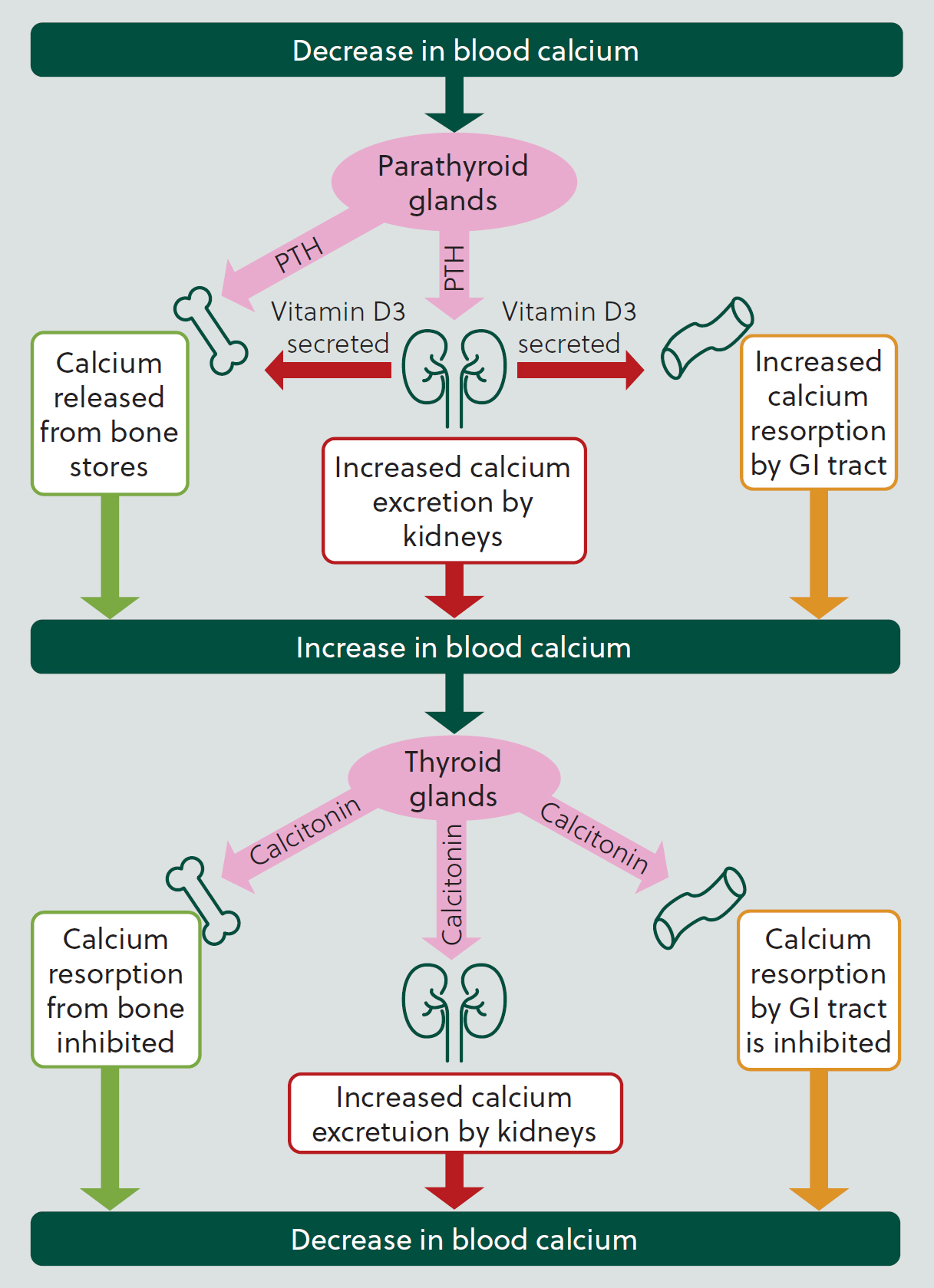
The largest calcium store in the body is bone, accounting for 99% of all calcium (Petroff and Greco, 2021). The remaining 1% is distributed between smaller stores in the extracellular and intracellular spaces (Figure 3) and a pool of soluble bone-stored calcium. Although the soluble bone pool of calcium is small (accounting for 0.5% of bone-stored calcium), it allows access to the larger insoluble bone pool, and as such plays an important role in calcium homeostasis.
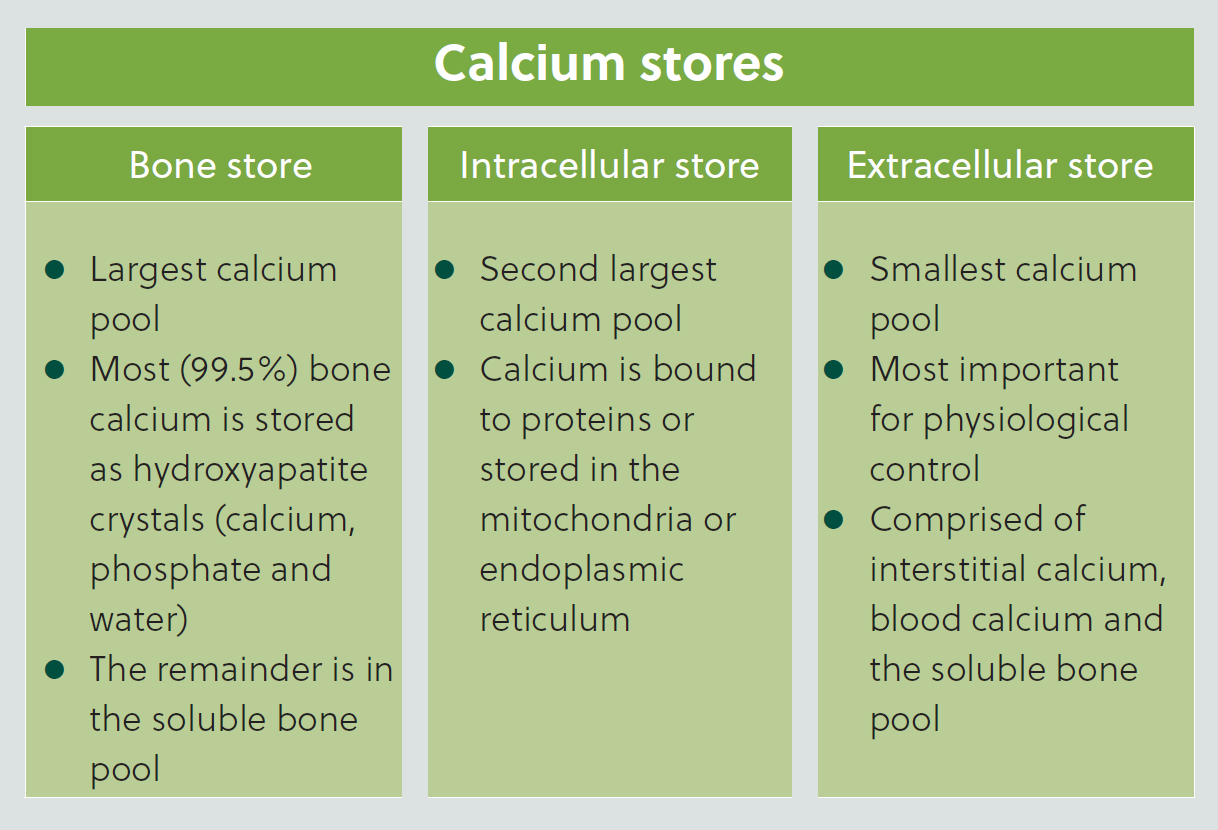
Hypocalcaemia
Subclinical hypocalcaemia
Subclinical hypocalcaemia is diagnosed when blood calcium concentration is below a pre-defined test cut-point associated with adverse outcomes, but clinical signs associated with low calcium are not evident; therefore, diagnosis can only be made based on biochemical results. The optimal cut-point for diagnosing subclinical hypocalcaemia is not universally agreed, and reported values range from 1.80‒2.35 mmol/L (Couto Serrenho et al, 2021), with some studies advocating lactation (ie age)-specific cut-points. Inconsistent results may be related to differences in study design and analytic methodology and readers are referred to a meta-analysis for a more detailed review (Couto Serrenho et al, 2021). Nevertheless, subclinical hypocalcaemia is known to be negatively associated with postpartum health and is reported to affect up to 60% of dairy cows after calving, with older cows being at higher risk (Reinhardt et al, 2011; Rodríguez et al, 2017; Venjakob et al, 2017; Neves et al, 2018). Accordingly, effective prevention of subclinical hypocalcaemia is as important as prevention of clinical hypocalcaemia.
Clinical hypocalcaemia
Clinical hypocalcaemia (also known as milk fever or parturient paresis) is diagnosed when blood calcium concentration is low and associated clinical signs are evident. In contrast to subclinical hypocalcaemia, the presence of characteristic clinical signs, signalment and a history of recent calving means that confirmatory biochemical results are not usually needed for diagnosis; however, it is good practice to obtain a pre-treatment blood sample from all cows with suspected hypocalcaemia for storage and testing at a later point if response to standard treatment is poor (McArt and Oetzel, 2023). Three distinct stages of clinical hypocalcaemia are described (Constable et al, 2017):
Treatment of clinical hypocalcaemia
Oral calcium treatment is recommended for cows presenting with stage one hypocalcaemia (ie cows that are still standing); however, if cows are recumbent, rapid treatment is needed to prevent secondary muscle and nerve injury. Standard treatment comprises the administration of a single bottle (400–500 mL) of calcium intravenously, accompanied by an additional bottle administered subcutaneously. This combined treatment approach is recommended because, while the clinical response to intravenous administration of calcium is fast and dependable in uncomplicated cases, intravenous administration of calcium is associated with transient hypercalcaemia (Blanc et al, 2014), inhibiting PTH release and stimulating calcitonin release. As a result, the cow stops mobilising calcium stores, gastrointestinal absorption of calcium reduces and renal excretion of calcium increases (Figure 2), causing a hypocalcaemic relapse 12–24 hours after treatment. Supplementation of intravenous calcium with subcutaneous or oral calcium treatment mitigates against this effect. Oral calcium treatment is preferred as this route leads to a more sustained and consistent blood calcium concentration for up to 48 hours after treatment (Blanc et al, 2014); if cows are recumbent or are unable to swallow effectively, subcutaneous calcium can be administered instead, but reduced peripheral perfusion may lessen the effectiveness of absorption.
When administering calcium intravenously, it also needs to be considered that rapid increases in blood calcium concentration can precipitate potentially fatal cardiac dysrhythmias, especially in cows that are endotoxic. To avoid this, slow intravenous administration of calcium is recommended, a rate of 1 g calcium per minute (typically 10–15 minutes for 400–500 mL of available products) has been suggested (Goff, 2008). Monitoring the cow’s heart rate and rhythm during administration is also advisable: if bradycardia or arrhythmias are heard, calcium administration should be stopped until a normal cardiac rate and rhythm are restored.
Prevention of hypocalcaemia
Nutritional management in the transition period is a commonly used strategy for preventing subclinical and clinical hypocalcaemia. The most common nutritional approaches are targeted to cows in the last three weeks of gestation and involve altering the dietary cation-anion balance or limiting the dietary calcium available for absorption. Calcium supplementation of cows immediately after calving is another common strategy that can be used alone or together with pre-calving nutritional management.
Manipulation of dietary cation-anion difference
Acid-base balance and electrical neutrality are the fundamental underlying principles of dietary cation-anion difference (DCAD) manipulation (Byers, 1993). Cations (positively charged ions) and anions (negatively charged ions) influence blood pH when absorbed into the bloodstream. Proportional increases in anions lead to a decrease in blood pH (ie more acidic), whereas increasing the proportion of cations leads to an increase in blood pH (ie more alkalotic or basic). To maintain electrical neutrality, when a mild acidosis is induced by increasing the relative proportion of anions in the bloodstream, the increase in negative charge needs to be buffered, which is achieved in part by the mobilisation of calcium ions (Ca2+) from bone stores and increased absorption of calcium from the gastrointestinal tract — increasing blood calcium concentration. When an acidogenic diet that contains a higher relative proportion of anions to cations (termed a negative DCAD) is consumed by cows a compensated metabolic acidosis is induced. If this type of diet is fed for the last three weeks of gestation, calcium is mobilised from bone stores before calving, preparing the cow for the high calcium demand of lactation and reducing hypocalcaemia risk. Additionally, it is thought that tissue responsiveness to PTH is increased under acidogenic conditions (Wilkens et al, 2020), further facilitating calcium mobilisation.
The beneficial effects of manipulation of the DCAD of a pre-calving ration on hypocalcaemia incidence have been described for 40 years (Block, 1984) and are well-studied. In the past two decades several meta-analyses have found that feeding a negative DCAD ration in late gestation is associated with increased postpartum blood calcium concentration and reduced hypocalcaemia risk (Charbonneau et al, 2006; Lean et al, 2006; Santos et al, 2019), as well as improved postpartum health (Lean et al, 2019; Santos et al, 2019). Available data suggest that multiparous cows may benefit the most from consuming a negative DCAD diet with research reporting potentially negative effects of feeding a negative DCAD diet to nulliparous cows (Santos et al, 2019); however, studies of nulliparous cows are few (one meta-analysis found only 8% of studied cows were nulliparous; Santos et al, 2019), and further research into the effects of feeding a negative DCAD ration to younger animals is warranted.
Acid-base metabolism is complex, and several equations have been proposed for the calculation of the DCAD of a ration (Lean et al, 2006) but as the DCAD is fundamentally a ratio of cations to anions, the simplest proposed equation that includes only the strong ions chloride (Cl−), sulphur (S2−), sodium (Na+), and potassium (K+) (Figure 4) is commonly used (Martín-Tereso and Martens, 2014). These ions are known as strong ions because they have the greatest influence on acid-base balance (Byers, 1993); although other (weaker) ions are involved in acid-base metabolism, their inclusion in DCAD calculations is thought to have little additional effect. Moreover, this equation is reported to be optimal for predicting hypocalcaemia risk (Lean et al, 2006).

Dietary cation-anion difference is usually measured in milliequivalents per kilogram of dry matter (mEq/kg DM), because it is a measure of electrical charge rather than mass (Byers, 1993).
To calculate DCAD, the figures input into the equation for each of the strong ions must be adjusted to reflect their electrical charge. This can be achieved by multiplying the proportion of each compound in the ration (% DM) by the relevant conversion factor (Table 1) (Constable et al, 2017). The combined equation taking this into account is presented in Figure 5.

Compound | Conversion factor |
---|---|
Sodium | 434.98 |
Potassium | 255.74 |
Chloride | 282.06 |
Sulphur | 623.75 |
The optimal DCAD for preventing hypocalcaemia is subject to debate, but it is well described that rations with a very negative DCAD are associated with reduced feed consumption. One meta-analysis concluded that it is likely that the DCAD of a dry cow ration does not need to be lower than −150 mEq/kg DM to prevent hypocalcaemia (Santos et al, 2019) effectively; however, some studies have suggested that exceeding −100 mEq/kg DM is of no benefit and may potentially be detrimental (Melendez and Poock, 2017; Lopera et al, 2018).
Limiting dietary calcium available for absorption
The preventative effect of feeding a low-calcium pre-calving diet has been known for several decades (Boda and Cole, 1954). Diets low in calcium (<20 g/cow/day) induce a state of mild hypocalcaemia that stimulates the secretion of PTH and increases blood calcium concentration through a combination of increased calcium mobilisation from bone, increased renal calcium resorption and enhanced efficiency of gastrointestinal calcium absorption (Goff, 2008). While the effectiveness of low-calcium pre-calving diets is well established experimentally, feeding a diet low enough in calcium to achieve the desired effect in practice proves challenging on most commercial dairy farms (Wilkens et al, 2020). Additionally, grass-based forages typically have a high potassium content, which can have an alkalinising effect because potassium is a positively charged strong ion that inhibits calcium mobilisation from bone stores and further impedes efforts to optimise blood calcium concentration in preparation for lactation. A common strategy used as an alternative to feeding low-calcium diets is the inclusion of calcium binders in the dry cow ration. The most widely studied calcium binder for this purpose is synthetic zeolite, Zeolite A (sodium aluminium silicate), and several Zeolite A products are commercially available. Zeolite A has been extensively studied in the past two decades, with studies consistently finding that cows fed Zeolite A for the last 2–3 weeks of gestation have higher blood calcium concentration at calving than control cows (Thilsing-Hansen et al, 2002; Kerwin et al, 2019; Frizzarini et al, 2024a). As such, it is now well accepted that the inclusion of Zeolite A in prepartum diets is an effective strategy for the prevention of hypocalcaemia.
Feeding Zeolite A is known to be associated with decreased serum phosphate and magnesium concentration at calving (Thilsing-Hansen et al, 2002; Kerwin et al, 2019; Frizzarini et al, 2024a), but this effect is transient and has not been found to be clinically significant. Inclusion of Zeolite A in the pre-calving ration has also been associated with a dose-dependent reduction in feed intake; therefore, very high doses should be avoided. Accordingly, current recommendations for feeding Zeolite A are to use at an inclusion rate of no more than 500 g/day for a maximum duration of 2 weeks (European Food Safety Authority, 2007).
How do negative DCAD and low calcium diets compare?
Few studies have compared nutritional strategies for preventing hypocalcaemia, but one study concluded that both negative DCAD diets or diets containing Zeolite A effectively enhance peri-partum calcium metabolism, likely through different mechanisms (Frizzarini et al, 2024a). Related work found that, while pre-partum dry matter intake (kg/d) and rumination time (min/d) of cows fed 500 g/d Zeolite A was lower than cows fed either a negative DCAD diet or control cows fed a positive DCAD diet, energy balance and postpartum health were unaffected (Frizzarini et al, 2024b). This supports the hypothesis that the suppressive effect on feed intake has limited clinical effect when Zeolite A is included in feed at recommended levels. Additionally, immunoglobulin G (IgG) concentration of colostrum harvested from cows in the Zeolite A group was higher than IgG concentration of colostrum from cows in the other two groups (Frizzarini et al, 2024b). This is an interesting finding that warrants further investigation. However, it should be noted that the IgG concentration of colostrum harvested from cows in all three groups exceeded the widely accepted quality threshold of 50 g/L (Godden et al, 2019) and the Brix measurement of colostrum quality did not differ between groups. Hence, the clinical importance of this result is as yet unclear.
Post-calving calcium supplementation
Although oral, subcutaneous, and intravenous routes can all be used for the administration of post-calving calcium treatment, oral administration is the preferred route. Blanc et al (2014) found serum calcium concentration of cows treated with intravenous calcium remained elevated for up to 4 hours after treatment, compared to 48 hours for cows treated with oral calcium. In contrast to intravenous administration, subcutaneous calcium treatment may elevate serum calcium concentration for 48 hours after treatment compared to cows not receiving any calcium (Amanlou et al, 2016), but data are conflicting, and other studies report effects lasting only 24 hours (Miltenburg et al, 2016). Subcutaneous calcium preparations can be irritant and may lead to tissue necrosis, so it is recommended to administer small volumes containing a maximum 1.5 g of calcium at multiple sites (Goff, 2008). For commercially available products in the UK (Mann et al, 2019), this equates to no more than 50 ml per site, which is impractical and may result in adverse effects associated with multiple injections; therefore, it is recommended that for routine prophylactic treatment of cows after calving oral calcium administration is used, typically in bolus form (Constable et al, 2017; McArt and Oetzel, 2023). Moreover, Jahani-Moghadam et al (2020) found that, while both oral and subcutaneous calcium administration elevate serum calcium concentration, oral calcium treatment may be more beneficial in the longer term, further supporting the recommendation to reserve subcutaneous preparations for treatment of clinical hypocalcaemia.
Multiparous cows have been found to benefit most from routine calcium administration at calving (Leno et al, 2018; Melendez et al, 2021), meaning older cows can be targeted for treatment, although studies of primiparous cows are few and more studies of young animals are needed (Valldecabres et al, 2023). Additionally, combining postpartum oral calcium treatment with feeding a pre-partum negative DCAD diet has been found to result in higher postpartum serum calcium concentration than using either strategy on its own (Afshar Farnia et al, 2018), supporting the common practice of using both approaches on farm.
Monitoring strategies
Urine pH measurement
To compensate for the metabolic acidosis induced by feeding a negative DCAD diet, sodium (Na+) and potassium (K+) ions are retained by the kidneys, altering the strong ion difference of urine and decreasing urine pH (Constable et al, 2009). Measurement of urine pH can be readily performed on farm using pH paper or a pH-meter (Constable et al, 2019); for accurate results, cows need to have consumed a negative DCAD ration for at least two days before testing urine pH (Goff, 2014; Caixeta and Omontese, 2021). The optimal target urine pH for cows fed a negative DCAD diet is subject to debate (Melendez and Chelikani, 2022). However, although some data support a target pH as low as 5.5 (Glosson et al, 2020; 2023), more moderate acidification resulting in a urine pH range of 6.0–7.0 is likely to be sufficient for effective prevention of hypocalcaemia (Charbonneau et al, 2006; Goff, 2014; Constable et al, 2019; Melendez and Chelikani, 2022). By contrast, it is well-accepted that urine pH values below 5.5 indicate that the DCAD of the ration is too low and indicates there is a risk cows will progress to uncompensated metabolic acidosis.
Assessment of urine pH is most valuable if performed regularly, as this enables trends to be monitored, and adjustment of the diet’s anion content can be performed quickly if required. As there can be a lot of between-cow variation in urine pH, it is recommended to evaluate the mean pH for each group tested, with sample sizes of at least eight cows recommended if possible (Cook et al, 2006).
Serum calcium concentration measurement
Measuring serum calcium concentration in the immediate postpartum period can offer valuable insight into the calcium status of the herd as cows affected with subclinical hypocalcaemia can be detected using this strategy. Sampling cows 12–24 hours postpartum is the classic approach (Cook et al, 2006); however, for approximately 20% of cows, subclinical hypocalcaemia at 24 hours after calving is transient and does not affect longer-term outcomes such as milk yield and postpartum health (McArt and Neves, 2020). By contrast, cows with hypocalcaemia at 4 days postpartum are at increased risk of adverse postpartum health events (McArt and Neves, 2020). Accordingly, measuring blood calcium concentration at 4 days after calving might be more representative of the calcium dynamics in postpartum cows and may have additional value in aiding farmers to identify cows that are more or less able to adapt to lactation. Blood testing each cow twice at 1 day and 4 days postpartum has been suggested to be optimal (McArt and Oetzel, 2023), but this approach can be challenging to implement due to the extra planning and effort needed compared to a single sample.
Conclusions
Clinical hypocalcaemia in dairy cows is a characteristic postpartum condition that is potentially life-threatening. Subclinical hypocalcaemia is more common than the clinical form but predisposes cows to poor postpartum health and adversely affects subsequent productivity, so recognition and management is crucial. Adoption of preventative strategies is key, but it is also important to have effective treatment protocols in place so that affected cows can be identified and treated promptly – reducing the risk of adverse outcomes.